Clinical significance of aetiological heterogeneity in classical Hodgkin lymphoma
Abstract
In this review we present contemporary understanding of aetiological heterogeneity in Hodgkin lymphoma, discuss how this may influence tumour phenotype and whether it does or may impact treatment outcomes. Many new treatments are being tested in this era. We especially discuss T-cell therapy and immune checkpoint blockade, because these two modern treatments are expected to have differential efficacy by the presence/absence of Epstein-Barr virus in the malignant Hodgkin-Reed-Sternberg cells. Survival after Hodgkin lymphoma is excellent in many patient strata with first-line treatment, but less so for patients with refractory or relapsing disease. On the other hand, this good prognosis also means that very large trials are needed to demonstrate superior efficacy of new treatment regimes. And our understanding of aetiological heterogeneity in Hodgkin lymphoma and how it affects prognosis is hampered for the same reason. We discuss the potential for fine-tuning risk stratification and treatment based on information that is little used today.
Keywords
INTRODUCTION
Hodgkin lymphoma (HL) is almost exclusively a B-cell malignancy annually diagnosed in an estimated 83,000 individuals worldwide[1]. The age-distribution of HL is unusual among cancers, by being bi- or tri-modal with a substantial number of cases in children, adolescents and young adults, and older adults, respectively[2]. Thus, HL spans the full spectrum from paediatric to geriatric malignancy and is believed to encompass entities of different aetiologies[2].
The treatment of HL is one of the success stories of modern oncology. The application of increasingly effective and gentle radiotherapy since the 1950s and subsequently combination chemotherapy and combined modality treatment have increased 5-year survival after HL from less than 10% to 90% or more today[3,4].
Today, most patients can potentially be cured even when diagnosed with advanced stage disease provided access to modern health care[3]. However, despite the generally favourable prognosis HL, both directly and indirectly, remains associated with increased morbidity and mortality. First, even with current state-of-the-art first-line treatment remission is not achieved for all patients and in other patients the disease relapses[5]. The prognosis for this non-negligible group of patients with refractory or relapsing disease, e.g., 15% of all HL patients in Sweden in the period 1992-2009, is considerably less favourable[3,4,6]. Secondly, follow-up studies of HL survivors continuously demonstrate a high frequency of a wide variety of adverse effects of the treatment and of the disease, accompanied by reduced quality of life and life expectancy[7-10]. E.g., not so long ago more early-stage HL patients died from long-term treatment complications, than from the lymphoma itself[3,4]. The excess morbidity and mortality, too, are particularly pronounced in the stratum of patients with relapsing or refractory disease[7,11].
Oncologists therefore face the difficult task of devising treatment strategies which simultaneously improve or maintain current HL cure rates and reduce the risk of long-term adverse effects of treatment among the survivors. Owing to the generally good outcomes achievable with current protocols there has been no strong incentive to investigate if even better results could be obtained by considering HL aetiology in treatment allocation.
However, this attitude may change following the successful introduction of novel treatment modalities into cHL treatment, which targets tumour cell characteristics and/or tumour cells’ interaction with the surrounding micro-environment, i.e., the tumour micro-environment (TME)[12]. The notion that aetiology would matter to HL treatment and treatment outcome hinges on the assumption that aetiologically heterogeneous variants of HL exist and that these exhibit differences that are therapeutically relevant.
Here, we provide a broad-brush picture aimed at motivating and pointing the way for renewed research into risk stratification considering HL aetiology. To do so in a concise and accessible way we have omitted many interesting details and viewpoints and deliberately used a compact set of references, making intensive use of a few other reviews.
CLASSIFICATION OF HODGKIN LYMPHOMA
The current WHO classification of tumours of haematopoietic and lymphoid tissues recognizes two immunophenotypically distinct HL variants, i.e., classical HL (cHL) and nodular lymphocyte predominant HL[13] of these, cHL constitutes some 95% of all HL cases[2]. The two types of HL are considered aetiologically, pathologically and clinically different[13], and in the following only cHL is considered.
HL lesions are histologically unusual among malignant diseases in that the neoplastic cells, the so-called Hodgkin/Reed-Sternberg (HRS) and their variants, constitute only a small fraction of the tumour lesion, typically a few percent in cHL[14]. Instead, the tumour lesions are entirely dominated by an admixture of inflammatory and accessory cells in which the malignant cells are scattered[14]. Based on the architecture of the TME, cHL is further divided into four subtypes, specifically mixed cellularity (MC), nodular sclerosis (NS), lymphocyte rich (LR) and lymphocyte depleted (LD) cHL[14]. The appreciation of these main histological types is important to the discussion of aetiological heterogeneity in cHL which rests primarily though not exclusively on epidemiological considerations.
Variation in HL incidence patterns suggests epidemiological heterogeneous variants
HL incidence varies by age both within and between populations[2]. Epidemiologically, the age-specific incidence distribution of HL in most settings has historically been construed as a mixture of two archetypical patterns, suggested to be characteristic of socio-economically affluent and deprived settings, respectively, in early survey data[15].
In affluent western countries age-specific cHL incidence tends to follow a bimodal distribution with separate peaks in adolescents and young adults (AYA) and in older adults, respectively. In deprived settings in contrast, there is no incidence peak in AYA but rather an incidence peak in younger children, which is then followed by an incidence peak in older adults[15].
It is implicitly understood from these associations that the age-specific incidence distributions are dynamic, especially in the first four decades of life[2,16]. Thus, in conjunction to societal development from deprived to an affluent setting an AYA HL incidence peak emerged, e.g., in Singapore[17]. Further illustrations of this phenomenon based on data from the United States, Slovenia, Finland, Estonia and Japan are discussed in Ref.[16].
Age, histology and tumour viral-status as markers of aetiologically distinct cHL variants
The conspicuous variation in age-specific incidence of HL along with other epidemiological and clinical differences fostered the first notion of aetiological heterogeneity in HL. Specifically, it was suggested that HL in children, in AYA, and in older adults were epidemiologically, possibly causally different[18,19].
To this day, epidemiological studies continuously consider age at diagnosis as an important characteristic to understand the natural history of cHL. Increasingly often, however, age at diagnosis is combined with either or both of two other features, tumour histology and tumour Epstein-Barr virus (EBV) status also suspected of defining aetiologically distinct cHL variants[2,16].
The incidence peak among AYA in affluent populations is mostly made up of NS cHL[2,16,20]. In contrast, MC cHL is more commonly seen in children and older adults, in socio-economically deprived settings and in patients with immune deficiencies[2,16,21]. In combination with other data pointing to different epidemiological characteristics it has therefore been suggested that MC and NS cHL represent aetiologically distinct entities[2,16,20].
Epstein-Barr virus and cHL
The contrasting incidence peaks among children in socio-economically deprived and among AYA in socio-economically affluent populations, respectively, led to speculations about HL being causally associated with a common childhood infectious agent whose oncogenic potential increases with age at exposure[15,22,23].
Here, the suspicion has been the strongest against EBV[24]. EBV is a ubiquitous lymphotropic human herpesvirus, which was first isolated from Burkitt lymphoma cell lines[25]. Depending on the socio-economic setting, primary infection with EBV typically occurs in early childhood (in deprived settings) or in adolescence (increasingly often in affluent settings), in which case it is then often accompanied by infectious mononucleosis[26]. Following primary infection, EBV latently infects B-lymphocytes to escape immune surveillance, with occasional lytic activations normally culled by the host’s immune system[27].
Following early demonstrations of EBV nucleic acids and gene products in malignant HRS cells in seminal papers in the late 1980s[28-31], we now know that only a subset of cHLs is EBV-positive and that the prevalence of EBV in cHL varies somewhat systematically with sex, age, and histology. Specifically, EBV prevalence is higher in cHLs diagnosed in males compared with females, in children and older adults compared with AYAs; in MC compared with NS cHL, and in deprived compared with affluent settings[21,32].
Importantly, although the prevalence of EBV in cHL correlates independently with both age at diagnosis and with histological types of cHL, none of the three captures the other two accurately and they likely represent different phenomena in the natural history of cHL[2]. Consequently, studies lacking any of the three characteristics may therefore be interpretationally challenging.
In addition to being biologically credible (see below), a variety of differences between EBV-positive and EBV-negative cHLs suggests that HRS EBV status distinguishes between aetiologically separate cHL entities[24]. For instance, epidemiological risk factors differ between EBV-positive and EBV-negative cHL. In particular, increased risks of HL following EBV-related infectious mononucleosis seem predominantly or even exclusively restricted to EBV-positive cHL as it is the case for the increased HL risk associated with immune deficiencies such as HIV infection and organ transplantation[2,16]. Other examples of exposures suspected of having different effects on the risk of EBV-positive and EBV-negative cHL include number of older and younger siblings and smoking[2,16]. These observations are supplemented by prospective serological analyses, which indicate that aberrant EBV-antibody patterns precede diagnosis of EBV-positive cHL, but not diagnosis of EBV-negative cHL[33].
Finally, genome-wide association studies have identified loci associated with risks of cHL irrespective of tumour EBV-status, as well as loci specific to EBV-positive and EBV-negative cHL (genetic studies are thoroughly reviewed and interpreted in Ref.[16]). Loci associated with cHL have been found both inside and outside of the HLA region and very strong associations between HLA-type and EBV-positive cHL have been known for a long time[34-37]. The association between some of the loci and cHL also varies by histology independently of tumour EBV status[38].
Nevertheless, neither HLA nor non-HLA loci has so far been used in treatment risk stratification and epidemiologically the most intriguing observation from genetic studies in HL to date may be the realisation that cHL has a much larger genetic overlap/similarity to autoimmune diseases than solid cancers[39].
In combination, these different lines of evidence have led to the formulation of a pathogenic model according to which EBV may lead to EBV-positive cHL either in direct continuation of the primary infection, be it in childhood or in AYA or because illness and/or immune senescence result in loss of immunological control of the normal latent stage of infection[24,40].
Traditional stratification of cHL treatment
First-line treatment of patients with cHL today generally consists of chemotherapy with or without radiotherapy, while patients with refractory or relapsing disease receive further chemotherapy and possibly autologous stem cell transplantation, and most recently also brentuximab vedotin, pembrolizumab and Nivolumab[6,41].
First-line treatment allocation is determined by stage of disease and scoring of established prognostic factors. Here, different staging systems have been used over time, most recently the Cotswolds modification of the Ann Arbor classification introduced in 1989, and the Lugano classification finalised in 2014[42]. Thus, depending on extent and location of disease patients are classified with early (stages I and II) or advanced-stage disease (stages III and IV). Staging is supplemented by different sets of characteristics known to have prognostic significance. In early-stage disease these factors include age, mediastinal tumour size, number of nodal sites and extra-nodal involvement, sedimentation rate, and the presence of specific - socalled B - symptoms[43,44]. For patients with advanced disease, characteristics influencing treatment allocation include age, stage, male sex, white blood cell count, lymphocyte and albumin and haemoglobin concentrations[43].
While patient survival was previously found to vary by tumour histology in univariate analyses[45] and it therefore was among prognostic factors in early-stage HL, it does not retain practical significance with modern treatment and in the presence of other prognostic factors using contemporary modelling techniques. Today it is therefore not considered by most centres and cooperative groups[43,44].
Tumour EBV status is not and has not previously been considered a prognostic factor in cHL treatment protocols. Presumably, the combination of a rare outcome (due to the good prognosis) in a rare disease and an assumed complicated association through interactions between tumour aetiology and outcome has made the gains from such analyses of tumour aetiology too uncertain to make the undertaking worthwhile in prospective studies. Also, histological and clinical presentation of cHL at diagnosis does not vary sufficiently by tumour EBV status to allow for inference from studies considering prognostic significance of clinical presentation alone[12,32].
Therefore, to evaluate the clinical significance of cHL EBV-status and histological subtype one must resort to retrospective studies and/or historical data concerning treatments no longer used. The bulk of such studies predating 2012 regarding EBV-status was recently reviewed and meta-analysed[32]. In combination, there was little to suggest from these studies that cHL outcome vary by tumour EBV-status whether with respect to event-free, disease-specific or overall survival[32]. While this could be due to methodological differences between studies [e.g., setting, study populations (trials, other), definition of cHL EBV status, types of treatment, etc.], it is also entirely plausible that clinical significance of cHL EBV status may vary between age groups considering the model for EBV-positive cHL pathogenesis. Such variation may easily escape detection in crude univariate meta-analyses, and on the other hand, may be hard to detect in suitably stratified analyses due to lack of statistical power.
Still, it is of some interest that albeit with some nuances, the three largest populations-based studies all suggested that compared with EBV-negative cHLs, EBV-positive cHLs may carry a better prognosis in younger adult patients and a worse prognosis in older adult patients, whether the outcomes considered were overall or disease-specific survival[46,47]. or failure-free and relative survival[48]. Patients included in the studies were diagnosed in 1988-2000 in the United States or Europe, but information on specific types of chemotherapy administered was reported in only one of these three studies where it did not vary by tumour EBV status[48]. Still, the lack of specific treatment information adds an additional layer of complications to the interpretation of the studies. Consequently, the literature is inconclusive with respect to potential difference in effect of frontline therapy (including ABVD and escBEACOPP) between EBV-positive and EBV-negative cHL.
EBV rescues pre-apoptotic germinal center cells
The scarcity of HRS cells in cHL tumour lesions for long hindered establishment of their cellular origin. However, in addition to being positive for the lymphocyte activation antigen CD30, genetic analyses revealed that most HRS cells carry clonal immunoglobulin chain gene rearrangements but are incapable of expressing immunoglobulins, implying that they are derived from pre-apoptotic germinal center B cells[49-51]. Despite their B-cell derivation the HRS cells do not express B-cell receptors which also would normally lead to their demise[52].
Though destined to undergo apoptosis, their continued survival and transformation into HRS cells implies that the cells at some point were rescued from the programmed apoptosis, which may be accomplished by EBV[53,54].
In EBV-positive HRS cells, EBV establishes a latency II program characterized by the expression of the viral proteins EBNA1, LMP1 and LMP2A and -2B along with EBERs and BART miRNA transcripts[53,55]. These viral gene products, most importantly LMP1, LMP2A and EBNA1, may substitute the cellular deficits to circumvent the pre-programmed apoptosis and to model the TME. LMP1 for instance mimics an activated CD40 receptor and stimulates the NF-κB, PI3-Akt and JAK/STAT pathway activity characteristic of HRS cells[55,56]. Moreover, in primary germinal center B-cells LMP1 has been shown to be capable of downregulating B-cell transcription factors and B-cell receptor signalling components and upregulate survival genes such as BCL2 and BFL-1[55,56]. LMP2A mimics the functions of an activated B-cell receptor and engages the RAS/PI3/AKT pathways critical of HRS cell survival[56]. LMP2A also suppresses the expression of transcription factors such as EBF1 and E2A and activates the Notch pathway[55,56]. EBNA1 in addition to sustaining viral and cellular gene expression and correct viral replication also contribute to HRS cell growth and survival through downregulation of the protein tyrosine phosphatase receptor type K (PTRPK)[55].
Lacking the virus but sharing fatal cellular characteristics with EBV-positive HRS cells, mechanisms that exert effects corresponding to those of EBV necessarily must exist to account for EBV-negative HRS cells. Although it has been speculated that EBV-negative HRS cells might initially have been EBV infected but lost the virus before completed transformation[57], other causal processes seem more likely. Thus, compared with EBV-positive HRS cells, EBV-negative HRS cells have significantly more cellular mutations, in particular in negative regulators of the NF-κB pathway such as TNFAIP3 and NFKBIA, more chromosomal breakpoints and aneuploid autosomes and almost never crippling immunoglobulin mutations[55].
EBV-positive cHL variants and modern treatment modalities
The generally poor prognosis of the non-negligible minority of patients with relapsed/refractory cHL has spurred the development of novel treatment modalities, which are devised based on growing understanding of the intricate biology of the interplay between the HRS cells and the TME. Complete reviews of cHL TME and of the array of novel treatment modalities for cHL lie outside the scope of the present paper, and interested readers are referred to Refs.[5,6,41,58].
Aetiological heterogeneity of cHL may be clinically relevant in the context of such novel cHL treatment approaches[6,12]. First, because EBV is present in the malignant cells in virus-positive cHL it makes sense to utilize this to target the HRS cells. Secondly, gene expression profiling suggest that the TME composition vary between EBV-positive and EBV-negative cHLs. Specifically, TME in EBV-positive cHLs is enriched for genes related to T-cell and antiviral activity compared with EBV-negative cHL[59].
One attempted novel treatment strategy taking advantage of EBV’s presence in the HRS cells aims to induce a lytic viral gene programme instead of the normal latency II pattern, which can be accomplished by, e.g., histone deacetylase inhibitors[60]. At the same time as the change to expression of lytic viral antigens per se should render the HRS cells more immunogenic to the host immune system, it would also provide a window of opportunity for the use of antiviral agents. For cHL this treatment strategy is still in its infancy and its full potential still to be established[61,62].
Among the other modern treatment strategies two attract particular interest, T-cell therapy and immune checkpoint blockade. These treatment approaches both depend on the effective presentation of antigens to the immune system. As mentioned, HLA class I and II constitution both seem important to cHL risk, but normal HLA-class I and HLA class II expression are commonly lost by the HRS cells, more so in EBV-negative cHL than in EBV-positive cHL[58]. Thus, Nijland et al.[63] recently investigated 361 cHL cases and reported HLA class I expression in 72.6% of EBV-positive cases as compared with 16.8% of EBV-negative cases, and HLA class II expression in 70.3% of EBV-positive cases and 53.2% of EBV-negative cases. Besides confirming previous reports of loss of HLA expression in HRS cells, Nijland et al.[63] also established that HLA class II expression would in effect be dysfunctional in many cases (38.8% of EBV-positive cases; 23.7% of EBV-negative cases).
Most EBV-positive HRS cells therefore should be able to present both EBV-derived antigenic peptides and neo-antigens and (therefore) also to elicit host immune-responses. The development of EBV-positive cHL is therefore a testament to the effectiveness of the immune-evasive mechanisms evoked by the HRS cells[58].
Despite these tumour-related mechanisms Bollard et al.[64] achieved good results with treatment with autologous expanded LMP-cytotoxic T-lymphocyte infusions in a study of 50 patients with EBV-positive lymphomas, half of which were cHLs.
Of the 25 patients with cHL with either active relapsing disease (n = 8) or in remission with high risk of relapse (n = 17), only four patients - all of whom had active disease - did not respond to treatment. Thus, at end of follow up 21 patients had achieved complete or sustained remission and were either free of cHL or had died for other reasons (five patients)[64].
Noteworthy, three of the eight patients with active disease had cHLs expressing the EBV latency III pattern typical of post-transplant lymphoproliferative diseases and all responded to treatment. In contrast, only one of the remaining five patients who exhibited the typical EBV latency II pattern responded to treatment, emphasizing the challenge of generating immune responses to latency type II antigens in HRS cells[64,65].
The group has continued their efforts to develop more effective EBV-specific T-cell therapy by making the T-cells resistant to the suppressive effect of TGF-β secreted by the TME. In a clinical trial including eight patients with or at risk of cHL relapse, two complete and one partial response was observed in seven patients who could be evaluated[66].
One Achilles’ heel to the original protocol used to generate EBV-specific autologous T-cells in the study reported by Bollard et al.[64] and Heslop et al.[67] was a rather lengthy production time - several months - during which the patient’s disease may progress. The group therefore revised their protocol and succeeded in generating autologous EBV-specific T-cells in a matter of weeks, that were seemingly equally effective in a small trial of 24 patients with type II latency EBV-positive cHL or non-Hodgkin lymphomas[67,68].
A further development in the use of EBV-specific T-cells has been trials using HLA-typed EBV-specific T-cells from donors[67]. So far, these trials have overwhelmingly concerned patients with post-transplant lymphoproliferative disease[67]. An extension of this idea is the ambition to develop off-the-shelf chimeric antigen receptor cells by transducing EBV-specific T-cells of relevance in the treatment of both EBV-positive and EBV-negative malignancies[69].
Whether or when EBV-specific T-cell therapy will eventually mature into a realistic treatment choice for cHL remains to be seen. This may be in combination with other treatment modalities analogous to Burkitt lymphoma, where a combination of hypomethylating agents inducing latency III programs in the malignant cells and EBV-specific T-cells has been proposed[70].
Interestingly, Bollard et al.[64] in their early study found that treatment response tended to be best for patients who had received T-cells with LMP1 specific activity and for patients in whom apparent epitope spreading to lymphoma antigens such as MAGE4, Survivin and PRAME was subsequently observed.
Recently, evidence to suggest that the presumed epitope spreading could be dependent on the induced LMP1 expression in B-cells were reported by Choi et al.[71]. Specifically, in a mouse model they demonstrated that LMP1 signalling in B-cells induced expression and presentation of tumour associated antigens on major histocompatibility complex classes I (MHC-I) and II (MHC-II) as well as upregulation of costimulatory ligands inducing potent cytotoxic (CD4+ and CD8+) T cell responses[71].
In principle, this could indicate that treatment with cytotoxic T-cells stimulated with LMP1 might also be effective in EBV-negative cHL, which do also express lymphoma antigens[72]. Indeed, in an additional experiment Choi et al.[71] showed that stimulation of autologous CD4 cells by CLL cells with induced LMP-1 expression produced cytotoxic T-cells capable of lysing CLL cells in vitro.
Besides suppression of HLA expression, one of the numerous mechanisms by which cHL HRS cells evade host immune response, is by expressing PD-L1/L2. This allows the HRS cells to interact with tumour-specific cytotoxic T-cells in the TME expressing the PD1, thereby effectively abrogating the T-cells’ activity[5].
The HRS cells’ overexpression of PD-L1 and PD-L2 is achieved by several different mechanisms including especially alterations of the 9p24.1 locus, which are seen in both EBV-negative and EBV-positive cHL[5,58,72].
However, for two reasons PD-L1 expression may be more pronounced in EBV-positive cHL than in EBV-negative cases. First, in EBV-positive, activation of the PA1 and JAK/STAT signalling pathway by LMP1 may increase PD-L1 expression[5,58]. Secondly, PD-L1 is also expressed by tumour-associated macrophages (TAM), which are more abundant in EBV-positive than in EBV-negative cHL TME[73], co-localise with
This communication between the HRS cells and the TME can be interfered with by antibodies blocking the interaction between PD-1 and PD-L1 and PD-L2, respectively. Two such compounds directed against PD1, Nivolumab and Pembrolizumab, in single-agent trials in relapsing and refractory cHL have produced overall response rates in the range of 54%-87%, but less impressive complete response rates (< 30%)[5].
Consequently, these drugs are now evaluated in combination with other treatments both as first-line treatment and for patients with relapsing or refractory disease[5,41]. Here, the combination of Nivolumab with brentuximab vedotin for relapsing or refractory cHL was recently reported to result in objective and complete response rates of 85% and 67%, respectively after a median follow-up of nearly three years[76].
The mechanisms by which PD-L1/L2 blockers induce tumour regression are still poorly understood. E.g., in one study HRS cells seemed to disappear almost instantaneously upon Nivolumab treatment initiation accompanied by decreased numbers of Type-1 regulatory T-cells and PD-L1-positive macrophages but not by clonal CD8-positive cytotoxic T-cell expansion[77]. Possibly the effects of Nivolumab treatment is in part mediated through newly recruited CD4-positive T-cell clones that are themselves cytotoxic and/or interact with innate immune cells, especially NK cells, which may correlate with treatment outcome[78]. Indeed, there is an increasing interest in understanding how HRS cells circumvent being culled by NK cells because of their loss of HLA class I[58,75,79,80] and in attempting to modulate/activate the innate immune response to treat cHL[81,82].
Theoretically treatment with PD-1 blockers[12,72] should result in better outcomes for EBV-positive cHLs than for EBV-negative cHLs; however, this cannot be determined based on currently reported studies, which also further details the rationale for these treatment proposals[5,83].
CONCLUSIONS
Epidemiologic studies have long suggested that EBV-positive and EBV-negative cHL are aetiologically different. With standard chemo- and radiotherapy for cHL the clinical relevance of this causal heterogeneity has been questionable. However, with modern modalities targeting HRS cell characteristics and determinants of TME, cHL aetiology may become important.
We have provided a simplified picture of this possibility but emphasize that there are other pathways and mechanisms as well that may be relevant for aetiology-specific cHL treatment. The TME is very dynamic and interacting with treatment regimens as well. Therefore, one cannot truly assess the efficacy of a certain combination of risk stratification algorithms and treatment modalities without conducting a large randomized trial designed for that purpose.
The introduction of T-cell therapy and immune checkpoint blockade into clinical practice in our view should motivate renewed ambitious efforts in developing proposals for risk stratification that at the outset aim at utilizing existing information, including histology and EBV-status, more comprehensively by applying modern statistical methods on all the available well-curated data. In all likelihood, the new treatment modalities described above and the underlying risk stratification schemes will turn out to be progress in HL treatment. Nevertheless it may be possible to do even better by using aetiological information more comprehensively in the risk stratification and treatment allocation, possibly at the minimal cost of introducing computer-assisted treatment allocation instead of the old simple and transparent risk scores.
DECLARATIONS
Authors’ contributionsReviewed papers and authored the paper together: Hjalgrim H, Rostgaard K
Availability of data and materialsNot applicable.
Financial support and sponsorshipNone.
Conflicts of interestBoth authors declared that there are no conflicts of interest.
Ethical approval and consent to participateNot applicable.
Consent for publicationNot applicable.
Copyright© The Author(s) 2022.
REFERENCES
1. Ferlay J, Ervik M, Lam FM, et al. Global and Regional Estimates of the Incidence and Mortality for 38 Cancers: GLOBOCAN 2018. Available from: https://gco.iarc.fr/today [Last accessed on 28 Jan 2022].
2. Hjalgrim H, Chang E, Glaser S. Hodgkin lymphoma. In: Thun MJ, Linet MS, Cerhan JR, Haiman CA, Schottenfeld D, editors. Cancer epidemiology and prevention. New York: Oxford University Press; 2018. p. 745-66.
3. Shanbhag S, Ambinder RF. Hodgkin lymphoma: a review and update on recent progress. CA Cancer J Clin 2018;68:116-32.
4. Biccler JL, Glimelius I, Eloranta S, et al. Relapse risk and loss of lifetime after modern combined modality treatment of young patients with Hodgkin lymphoma: a Nordic lymphoma epidemiology group study. J Clin Oncol 2019;37:703-13.
5. Caro J, Diefenbach C. New approaches to managing relapsed/refractory Hodgkin lymphoma: the role of checkpoint inhibitors and beyond. Expert Rev Hematol 2021;14:741-50.
6. Glimelius I, Diepstra A. Novel treatment concepts in Hodgkin lymphoma. J Intern Med 2017;281:247-60.
7. Glimelius I, Ekberg S, Jerkeman M, et al. Long-term survival in young and middle-aged Hodgkin lymphoma patients in Sweden 1992-2009-trends in cure proportions by clinical characteristics. Am J Hematol 2015;90:1128-34.
8. Ng AK, van Leeuwen FE. Hodgkin lymphoma: late effects of treatment and guidelines for surveillance. Semin Hematol 2016;53:209-15.
9. Björkholm M, Weibull CE, Eloranta S, Smedby KE, Glimelius I, Dickman PW. Greater attention should be paid to developing therapies for elderly patients with Hodgkin lymphoma-A population-based study from Sweden. Eur J Haematol 2018;101:106-14.
10. Glimelius I, Eloranta S, Ekberg S, Chang ET, Neovius M, Smedby KE. Increased healthcare use up to 10 years among relapse-free Hodgkin lymphoma survivors in the era of intensified chemotherapy and limited radiotherapy. Am J Hematol 2017;92:251-8.
11. Glimelius I, Englund A, Rostgaard K, et al. Distribution of hospital care among pediatric and young adult Hodgkin lymphoma survivors-A population-based cohort study from Sweden and Denmark. Cancer Med 2019;8:4918-27.
12. Carbone A, Gloghini A, Carlo-Stella C. Are EBV-related and EBV-unrelated Hodgkin lymphomas different with regard to susceptibility to checkpoint blockade? Blood 2018;132:17-22.
13. Swerdlow SH, Campo E, Pileri SA, et al. The 2016 revision of the World Health Organization classification of lymphoid neoplasms. Blood 2016;127:2375-90.
14. Stein H, Delsol G, Pileri SA, Weiss LM, Poppema S, Jaffe ES. Classical Hodgkin lymphoma, introduction, In: Swerdlow SH, Campo E, Harris NL, Jaffe ES, Pileri SA, Stein H, Thiele J, Vardiman JW, editors. WHO classification of tumours of haematopoietic and lymphoid tissues. Lyon, France: International Agency for Research on Cancer; 2008. p. 326-9.
16. Cozen W, Kavianpour B, Mack TM. The epidemiology of Hodgkin lymphoma. In: Hudnall S, Küppers R, editors. Precision molecular pathology of Hodgkin lymphoma. Springer International Publishing; 2017. p. 157-96.
17. Hjalgrim H, Seow A, Rostgaard K, Friborg J. Changing patterns of Hodgkin lymphoma incidence in Singapore. Int J Cancer 2008;123:716-9.
18. Macmahons B. Epidemiological evidence on the nature of Hodgkin’s disease. Cancer 1957;10:1045-54.
20. Cozen W, Katz J, Mack TM. Risk patterns of Hodgkin’s disease in Los Angeles vary by cell type. Cancer Epidemiol Biomarkers Prev 1992;1:261-8.
21. Glaser SL, Lin RJ, Stewart SL, et al. Epstein-Barr virus-associated Hodgkin’s disease: epidemiologic characteristics in international data. Int J Cancer 1997;70:375-82.
22. Gutensohn N, Cole P. Epidemiology of Hodgkin’s disease in the young. Int J Cancer 1977;19:595-604.
25. Epstein M, Achong B, Barr Y. Virus particles in cultured lymphoblasts from Burkitt’s lymphoma. Lancet 1964;283:702-3.
26. Rostgaard K, Balfour HH Jr, Jarrett R, et al. Primary Epstein-Barr virus infection with and without infectious mononucleosis. PLoS One 2019;14:e0226436.
27. Thorley-Lawson DA. Epstein-Barr virus: exploiting the immune system. Nat Rev Immunol 2001;1:75-82.
28. Poppema S, van Imhoff G, Torensma R, Smit J. Lymphadenopathy morphologically consistent with Hodgkin’s disease associated with Epstein-Barr virus infection. Am J Clin Pathol 1985;84:385-90.
29. Weiss LM, Strickler JG, Warnke RA, Sklar J. Epstein-Barr viral DNA in tissues of Hodgkin’s disease. Am J Pathology 1987;129:86-91.
30. Anagnostopoulos I, Herbst H, Niedobitek G, Stein H. Demonstration of monoclonal EBV genomes in Hodgkin’s disease and Ki-1-positive anaplastic large cell lymphoma by combined Southern blot and in situ hybridization. Blood 1989;74:810-6.
31. Weiss LM, Movahed LA, Warnke RA, Sklar J. Detection of Epstein-Barr viral genomes in Reed-Sternberg cells of Hodgkin’s disease. N Engl J Med 1989;320:502-6.
32. Lee JH, Kim Y, Choi JW, Kim YS. Prevalence and prognostic significance of Epstein-Barr virus infection in classical Hodgkin’s lymphoma: a meta-analysis. Arch Med Res 2014;45:417-31.
33. Levin LI, Chang ET, Ambinder RF, et al. Atypical prediagnosis Epstein-Barr virus serology restricted to EBV-positive Hodgkin lymphoma. Blood 2012;120:3750-5.
34. Diepstra A, Niens M, Vellenga E, et al. Association with HLA class I in Epstein-Barr-virus-positive and with HLA class III in Epstein-Barr-virus-negative Hodgkin’s lymphoma. Lancet 2005;365:2216-24.
35. Enciso-Mora V, Broderick P, Ma Y, et al. A genome-wide association study of Hodgkin’s lymphoma identifies new susceptibility loci at 2p16.1 (REL), 8q24.21 and 10p14 (GATA3). Nat Genet 2010;42:1126-30.
36. Urayama KY, Jarrett RF, Hjalgrim H, et al. Genome-wide association study of classical Hodgkin lymphoma and Epstein-Barr virus status-defined subgroups. J Natl Cancer Inst 2012;104:240-53.
37. Cozen W, Timofeeva MN, Li D, et al. A meta-analysis of Hodgkin lymphoma reveals 19p13.3 TCF3 as a novel susceptibility locus. Nat Commun 2014;5:3856.
38. Delahaye-Sourdeix M, Urayama KY, Gaborieau V, et al. A novel risk locus at 6p21.3 for Epstein-Barr virus-positive Hodgkin lymphoma. Cancer Epidemiol Biomarkers Prev 2015;24:1838-43.
39. Khankhanian P, Cozen W, Himmelstein DS, et al. Meta-analysis of genome-wide association studies reveals genetic overlap between Hodgkin lymphoma and multiple sclerosis. Int J Epidemiol 2016;45:728-40.
41. Desai S, Ansell SM. Future directions in Hodgkin lymphoma: checkpoint inhibitors and beyond. Leuk Lymphoma 2021;62:1795-804.
42. Cheson BD, Fisher RI, Barrington SF, et al. Alliance, Australasian Leukaemia and Lymphoma Group, Eastern Cooperative Oncology Group, European Mantle Cell Lymphoma Consortium, Italian Lymphoma Foundation, European Organisation for Research, Treatment of Cancer/Dutch Hemato-Oncology Group, Grupo Español de Médula Ósea, German High-Grade Lymphoma Study Group, German Hodgkin’s Study Group, Japanese Lymphorra Study Group, Lymphoma Study Association, NCIC Clinical Trials Group, Nordic Lymphoma Study Group, Nordic Lymphoma Study Group, United Kingdom National Cancer Research Institute. Recommendations for initial evaluation, staging, and response assessment of Hodgkin and non-Hodgkin lymphoma: the Lugano classification. J Clin Oncol 2014;32:3059-68.
43. Ansell SM. Hodgkin lymphoma: 2016 update on diagnosis, risk-stratification, and management. Am J Hematol 2016;91:434-42.
44. Bröckelmann PJ, Specht L. Prognostic Factors. In: Engert A, Younes A, editors. Hodgkin lymphoma - a comprehensive review. Cham, Switzerland: Springer Nature Switzerland AG; 2020. p. 145-70.
45. Allemani C, Sant M, De Angelis R, Marcos-Gragera R, Coebergh JW. EUROCARE Working Group. Hodgkin disease survival in Europe and the U.S.: prognostic significance of morphologic groups. Cancer 2006;107:352-60.
46. Keegan TH, Glaser SL, Clarke CA, et al. Epstein-Barr virus as a marker of survival after Hodgkin’s lymphoma: a population-based study. J Clin Oncol 2005;23:7604-13.
47. Jarrett RF, Stark GL, White J, et al. Scotland and Newcastle Epidemiology of Hodgkin Disease Study Group. Impact of tumor Epstein-Barr virus status on presenting features and outcome in age-defined subgroups of patients with classic Hodgkin lymphoma: a population-based study. Blood 2005;106:2444-51.
48. Diepstra A, van Imhoff GW, Schaapveld M, et al. Latent Epstein-Barr virus infection of tumor cells in classical Hodgkin’s lymphoma predicts adverse outcome in older adult patients. J Clin Oncol 2009;27:3815-21.
49. Küppers R, Rajewsky K, Zhao M, et al. Hodgkin disease: Hodgkin and Reed-Sternberg cells picked from histological sections show clonal immunoglobulin gene rearrangements and appear to be derived from B cells at various stages of development. Proc Natl Acad Sci U S A 1994;91:10962-6.
50. Kanzler H, Küppers R, Hansmann ML, Rajewsky K. Hodgkin and Reed-Sternberg cells in Hodgkin’s disease represent the outgrowth of a dominant tumor clone derived from (crippled) germinal center B cells. J Exp Med 1996;184:1495-505.
51. Marafioti T, Hummel M, Foss HD, et al. Hodgkin and reed-sternberg cells represent an expansion of a single clone originating from a germinal center B-cell with functional immunoglobulin gene rearrangements but defective immunoglobulin transcription. Blood 2000;95:1443-50.
52. Mancao C, Altmann M, Jungnickel B, Hammerschmidt W. Rescue of “crippled” germinal center B cells from apoptosis by Epstein-Barr virus. Blood 2005;106:4339-44.
53. Jarrett RF, Hjalgrim H, Murray PG. The role of viruses in the genesis of Hodgkin lymphoma. In: Engert A, Younes A, editors. Hodgkin lymphoma - a comprehensive review. Cham: Springer; 2018. p. 25-46.
54. Rosenwald A, Küppers R. Pathology and molecular pathology of Hodgkin lymphoma. In: Engert A, Younes A, editors. Hodgkin lymphoma - a comprehensive overview, Cham: Springer; 2020. p. 47-68.
55. Murray PG, Young LS. An etiological role for the Epstein-Barr virus in the pathogenesis of classical Hodgkin lymphoma. Blood 2019;134:591-6.
56. Murray P, Ibrahim M. Role of EBV in classical Hodgkin lymphoma. In: Hudnall SD, Küppers R, editors. Precision molecular pathology of Hodgkin lymphoma. Cham: Springer; 2018. p. 91-110.
58. Visser L, Veldman J, Poppema S, van den Berg A, Diepstra A. Microenvironment, cross-talk, and immune escape mechanisms. In: Engert A, Younes A, editors. Hodgkin lymphoma - a comprehensive overview. Cham: Springer; 2020. p. 69-86.
59. Chetaille B, Bertucci F, Finetti P, et al. Molecular profiling of classical Hodgkin lymphoma tissues uncovers variations in the tumor microenvironment and correlations with EBV infection and outcome. Blood 2009;113:2765-3775.
60. Ghosh SK, Perrine SP, Williams RM, Faller DV. Histone deacetylase inhibitors are potent inducers of gene expression in latent EBV and sensitize lymphoma cells to nucleoside antiviral agents. Blood 2012;119:1008-17.
61. Porcu P, Haverkos B, Alpdogan O, et al. Oral nanatinostat (Nstat) and valganciclovir (VGCV) in patients with recurrent Epstein-Barr virus (EBV)-positive lymphomas: initial phase 2 results. Blood 2020;136:7-8.
62. Perrine SP, Hermine O, Small T, et al. A phase 1/2 trial of arginine butyrate and ganciclovir in patients with Epstein-Barr virus-associated lymphoid malignancies. Blood 2007;109:2571-8.
63. Nijland M, Veenstra RN, Visser L, et al. HLA dependent immune escape mechanisms in B-cell lymphomas: implications for immune checkpoint inhibitor therapy? Oncoimmunology 2017;6:e1295202.
64. Bollard CM, Gottschalk S, Torrano V, et al. Sustained complete responses in patients with lymphoma receiving autologous cytotoxic T lymphocytes targeting Epstein-Barr virus latent membrane proteins. J Clin Oncol 2014;32:798-808.
65. Ho C, Ruella M, Levine BL, Svoboda J. Adoptive T-cell therapy for Hodgkin lymphoma. Blood Adv 2021;5:4291-302.
66. Bollard CM, Tripic T, Cruz CR, et al. Tumor-specific T-cells engineered to overcome tumor immune evasion induce clinical responses in patients with relapsed hodgkin lymphoma. J Clin Oncol 2018;36:1128-39.
67. Heslop HE, Sharma S, Rooney CM. Adoptive T-cell therapy for Epstein-Barr virus-related lymphomas. J Clin Oncol 2021;39:514-24.
68. Rouce RH, Mehta B, Sharma S, et al. Rapidly-generated EBV-specific T cells (EBVST-cells) to treat type 2 latency lymphoma. Blood 2016;128:2990.
69. Curran KJ, Sauter CS, Kernan NA, et al. Durable remission following “off-the-shelf” chimeric antigen receptor (CAR) T-cells in patients with relapse/refractory (R/R) B-cell malignancies. Biol Blood Marrow Tr 2020;26:S89.
70. Dalton T, Doubrovina E, Pankov D, et al. Epigenetic reprogramming sensitizes immunologically silent EBV+ lymphomas to virus-directed immunotherapy. Blood 2020;135:1870-81.
71. Choi IK, Wang Z, Ke Q, et al. Mechanism of EBV inducing anti-tumour immunity and its therapeutic use. Nature 2021;590:157-62.
72. Mottok A, Steidl C. Biology of classical Hodgkin lymphoma: implications for prognosis and novel therapies. Blood 2018;131:1654-65.
73. Kamper P, Bendix K, Hamilton-Dutoit S, Honoré B, Nyengaard JR, d’Amore F. Tumor-infiltrating macrophages correlate with adverse prognosis and Epstein-Barr virus status in classical Hodgkin’s lymphoma. Haematologica 2011;96:269-76.
74. Guo B, Cen H, Tan X, Ke Q. Meta-analysis of the prognostic and clinical value of tumor-associated macrophages in adult classical Hodgkin lymphoma. BMC Med 2016;14:159.
75. Vari F, Arpon D, Keane C, et al. Immune evasion via PD-1/PD-L1 on NK cells and monocyte/macrophages is more prominent in Hodgkin lymphoma than DLBCL. Blood 2018;131:1809-19.
76. Advani RH, Moskowitz AJ, Bartlett NL, et al. Brentuximab vedotin in combination with nivolumab in relapsed or refractory Hodgkin lymphoma: 3-year study results. Blood 2021;138:427-38.
77. Reinke S, Bröckelmann PJ, Iaccarino I, et al. Tumor and microenvironment response but no cytotoxic T-cell activation in classic Hodgkin lymphoma treated with anti-PD1. Blood 2020;136:2851-63.
78. Cader FZ, Hu X, Goh WL, et al. A peripheral immune signature of responsiveness to PD-1 blockade in patients with classical Hodgkin lymphoma. Nat Med 2020;26:1468-79.
79. Veldman J, Visser L, Berg AVD, Diepstra A. Primary and acquired resistance mechanisms to immune checkpoint inhibition in Hodgkin lymphoma. Cancer Treat Rev 2020;82:101931.
80. Diepstra A, Poppema S, Boot M, et al. HLA-G protein expression as a potential immune escape mechanism in classical Hodgkin’s lymphoma. Tissue Antigens 2008;71:219-26.
81. Bartlett NL, Herrera AF, Domingo-Domenech E, et al. A phase 1b study of AFM13 in combination with pembrolizumab in patients with relapsed or refractory Hodgkin lymphoma. Blood 2020;136:2401-9.
82. Csizmar CM, Ansell SM. Engaging the innate and adaptive antitumor immune response in lymphoma. Int J Mol Sci 2021;22:3302.
Cite This Article
Export citation file: BibTeX | RIS
OAE Style
Hjalgrim H, Rostgaard K. Clinical significance of aetiological heterogeneity in classical Hodgkin lymphoma. J Transl Genet Genom 2022;6:134-46. http://dx.doi.org/10.20517/jtgg.2021.46
AMA Style
Hjalgrim H, Rostgaard K. Clinical significance of aetiological heterogeneity in classical Hodgkin lymphoma. Journal of Translational Genetics and Genomics. 2022; 6(1): 134-46. http://dx.doi.org/10.20517/jtgg.2021.46
Chicago/Turabian Style
Hjalgrim, Henrik, Klaus Rostgaard. 2022. "Clinical significance of aetiological heterogeneity in classical Hodgkin lymphoma" Journal of Translational Genetics and Genomics. 6, no.1: 134-46. http://dx.doi.org/10.20517/jtgg.2021.46
ACS Style
Hjalgrim, H.; Rostgaard K. Clinical significance of aetiological heterogeneity in classical Hodgkin lymphoma. J. Transl. Genet. Genom. 2022, 6, 134-46. http://dx.doi.org/10.20517/jtgg.2021.46
About This Article
Special Issue
Copyright
Data & Comments
Data
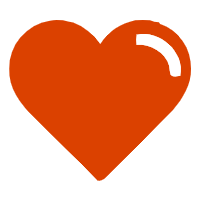

Comments
Comments must be written in English. Spam, offensive content, impersonation, and private information will not be permitted. If any comment is reported and identified as inappropriate content by OAE staff, the comment will be removed without notice. If you have any queries or need any help, please contact us at support@oaepublish.com.